A. Feizpour1,2, V. Doré2,3, J.D. Doecke4, Z.S. Saad5, G. Triana-Baltzer5, R. Slemmon5, P. Maruff6, N. Krishnadas1,2, P. Bourgeat4, K. Huang2, C. Fowler1, S.R. Rainey-Smith7,8,9, A.I. Bush1, L. Ward1, J. Robertson1, R.N. Martins8,9, C.L. Masters1, V.L. Villemagne2,10, J. Fripp4, H.C. Kolb5, C.C. Rowe1,2,11
1. The Florey Institute of Neuroscience and Mental Health, The University of Melbourne, Parkville, Victoria, Australia; 2. Department of Molecular Imaging & Therapy, Austin Health, Melbourne, Victoria, Australia; 3. The Australian e-Health Research Centre, CSIRO, Melbourne, Victoria, Australia; 4. The Australian e-Health Research Centre, CSIRO, Brisbane, Queensland, Australia; 5. Neuroscience Biomarkers, Janssen Research and Development, La Jolla, CA, USA; 6. Cogstate Ltd, Melbourne, VIC, Australia; 7. Centre for Healthy Ageing, Health Futures Institute, Murdoch University, Murdoch, Western Australia, Australia; 8. Edith Cowan University, Perth, Australia; 9. Australian Alzheimer’s Research Foundation, Nedlands, Perth, Australia; 10. Department of Psychiatry, University of Pittsburgh, Pittsburgh, PA, USA; 11. Florey Department of Neuroscience and Mental Health, The University of Melbourne, Melbourne, Victoria, Australia.
Corresponding Author: Professor Christopher C Rowe, Department of Molecular Imaging & Therapy, Austin Health, 145 Studley Road, Heidelberg, VIC. 3084, Australia. Telephone: +61-3-9496 3321. Fax +61-3-9458 5023. Email: christopher.rowe@austin.org.au
J Prev Alz Dis 2023;4(10):828-836
Published online July 5, 2023, http://dx.doi.org/10.14283/jpad.2023.83
Abstract
BACKGROUND: Plasma p217+tau has shown high concordance with cerebrospinal fluid (CSF) and positron emission tomography (PET) measures of amyloid-β (Aβ) and tau in Alzheimer’s Disease (AD). However, its association with longitudinal cognition and comparative performance to PET Aβ and tau in predicting cognitive decline are unknown.
OBJECTIVES: To evaluate whether p217+tau can predict the rate of cognitive decline observed over two-year average follow-up and compare this to prediction based on Aβ (18F-NAV4694) and tau (18F-MK6240) PET. We also explored the sample size required to detect a 30% slowing in cognitive decline in a 2-year trial and selection test cost using p217+tau (pT+) as compared to PET Aβ (A+) and tau (T+) with and without p217+tau pre-screening.
DESIGN: A prospective observational cohort study.
SETTING: Participants of the Australian Imaging, Biomarker & Lifestyle Flagship Study of Ageing (AIBL) and Australian Dementia Network (ADNeT).
PARTICIPANTS: 153 cognitively unimpaired (CU) and 50 cognitively impaired (CI) individuals.
MEASUREMENTS: Baseline p217+tau Simoa® assay, 18F-MK6240 tau-PET and 18F-NAV4694 Aβ-PET with neuropsychological follow-up (MMSE, CDR-SB, AIBL-PACC) over 2.4 ± 0.8 years.
RESULTS: In CI, p217+tau was a significant predictor of change in MMSE (β = -0.55, p < 0.001) and CDR-SB (β =0.61, p < 0.001) with an effect size similar to Aβ Centiloid (MMSE β = -0.48, p = 0.002; CDR-SB β = 0.43, p = 0.004) and meta-temporal (MetaT) tau SUVR (MMSE: β = -0.62, p < 0.001; CDR-SB: β = 0.65, p < 0.001). In CU, only MetaT tau SUVR was significantly associated with change in AIBL-PACC (β = -0.22, p = 0.008). Screening pT+ CI participants into a trial could lead to 24% reduction in sample size compared to screening with PET for A+ and 6-13% compared to screening with PET for T+ (different regions). This would translate to an 81-83% biomarker test cost-saving assuming the p217+tau test cost one-fifth of a PET scan. In a trial requiring PET A+ or T+, p217+tau pre-screening followed by PET in those who were pT+ would cost more in the CI group, compared to 26-38% biomarker test cost-saving in the CU.
CONCLUSION: Substantial cost reduction can be achieved using p217+tau alone to select participants with MCI or mild dementia for a clinical trial designed to slow cognitive decline over two years, compared to participant selection by PET. In pre-clinical AD trials, p217+tau provides significant cost-saving if used as a pre-screening measure for PET A+ or T+ but in MCI/mild dementia trials this may add to cost both in testing and in the increased number of participants needed for testing.
Key words: Blood based biomarkers, cognition, clinical trials, Alzheimer’s disease, pTau.
Introduction
The research framework proposed by the National Institute on Aging and the Alzheimer’s Association (NIA-AA) (1) reflects the shift in the definition of Alzheimer’s disease (AD) from a syndromal to a biological construct. This shift is also noticed in recent therapeutic trials that rely on a combination of clinical diagnosis, neuropsychological and clinical assessments, genetic risk factors and neuroimaging and fluid biomarker techniques for participant selection. Although well-established biomarkers such as cerebrospinal fluid (CSF) and molecular neuroimaging biomarkers can provide critical insight into the biological foundation of AD and have proven diagnostic utility, blood biomarkers provide better scalability, simplicity and cost reduction for widespread use.
Plasma phosphorylated tau (p-tau) biomarkers are promising candidates with more than 30 potential phosphorylation sites. Recent work has shown that among the currently known p-tau biomarkers, the ones phosphorylated at threonine 181, 217 or 231 reflect brain tau and amyloid-β (Aβ) pathophysiology (2). Ultra-High-sensitivity detection immunoassay systems such as Meso Scale Discovery (MSD) and single molecule array (Simoa) have enabled assay of ptau species in plasma including p-tau181 (3, 4), p-tau217 (5, 6), p-tau231 (6, 7). Plasma p-tau181 has been the most widely used p-tau biomarker, showing a strong association with both Aβ and tau positron emission tomography (PET) (4), enabling differential diagnosis of AD dementia from other neurodegenerative diseases (8, 9) and prediction of future cognitive decline (3).
More recently, a novel plasma p-tau217 measure— p217+tau— was developed that detects tau phosphorylation at threonine 217 and is augmented by phosphorylation at threonine 212. Tau in neurofibrillary tangles in AD is phosphorylated at multiple sites (10), so dual epitope pT217 plus pT212 detection may have advantages over single site p-tau measures. P217+tau has shown similar diagnostic performance to that of p-tau217 and high predictive accuracy for CSF and PET Aβ and tau status (11). It has also been shown to elevate early in the preclinical phase of the AD continuum, discriminate Aβ- cognitively unimpaired (CU) from Aβ+ AD individuals with area under the receiver operating characteristic curve (AUC) of 0.94 and AD from other dementia with AUC of 0.93 (12).
However, the association of p217+tau with longitudinal cognition and its comparative performance to neuroimaging biomarkers of tau and Aβ in predicting prospective cognitive decline has not yet been investigated. This information is essential to estimate the impact of plasma p217+ tau trial screening on sample size, trial duration and cost of disease modifying clinical trials in AD.
In this study, we investigated plasma p217+tau in the Australian Imaging, Biomarker & Lifestyle Flagship Study of Ageing (AIBL) and Australian Dementia Network (ADNeT) cohorts. We examined whether p217+tau can 1) predict prospective rate of cognitive decline on multiple well-established measures of cognition; 2) be a comparable predictor of cognitive decline to neuroimaging biomarkers of Aβ (18F-NAV4694) and tau (18F-MK6240); and 3) provide a (pre)screening strategy to decrease the cost of therapeutic trials aiming to slow cognitive decline. All objectives were investigated in a CU group and a cognitively impaired (CI) group to assess performance of p217+tau in early and later stages of the AD continuum.
Methods
Participants
A total of 397 participants from the AIBL and ADNeT cohorts with baseline 18F-MK6240 tau PET, 18F-NAV4694 Aβ PET and plasma p217+tau was initially included. Cohort recruitment and evaluation are detailed in a previous report (13). All participants were clinically classified by a multi-disciplinary panel, blind to PET imaging and blood assays results, as cognitively unimpaired (CU) or cognitively impaired (CI) with mild cognitive impairment (MCI), Alzheimer’s disease (AD) dementia or non-AD dementia. The requirement for a diagnosis of CU included performance within 1.5 standard deviations (SD) of the published norms for their age group on selected neuropsychological assessments. Internationally agreed criteria were used for a diagnosis of MCI (14) or AD dementia (15). To calculate the prevalence of biomarker positivity in CU and CI respectively, all 397 participants (225 CU, 172 CI) were included. Of the 397 participants, 203 (153 CU, 29 MCI and 21 mild AD dementia) had one or more follow-up neuropsychological assessments and were included in all other analyses using longitudinal data. Approval was obtained from institutional ethical review committees for the AIBL and ADNeT studies and written informed consent were obtained from all participants.
Neuropsychological and Clinical Assessment
All participants had the AIBL neuropsychology battery administered, as previously described in detail (16). To investigate the yearly rate of change in cognition, three measures were used: 1) Mini-Mental State Examination (MMSE); 2) Clinical Dementia Rating Sum of Boxes (CDR-SB) which is a validated measure of dementia severity (17); 3) AIBL Preclinical Alzheimer’s disease Cognitive Composite (AIBL-PACC). The AIBL-PACC is based on the Alzheimer Disease Cooperative Study (ADCS)-PACC (18) and is known to be sensitive to cognitive deterioration in clinically normal older adults. The AIBL-PACC consists of the MMSE, Digit Symbol Substitution Test from the Wechsler Adult Intelligence Scale, California Verbal Learning Test II long delay free recall, and Logical Memory IIa subtest from the Wechsler Memory Scale. Neuropsychological and clinical assessments were performed at baseline and follow-up(s). Average time of follow up(s) was 2.4 ± 0.8 years from baseline.
Image Acquisition and Analysis
Aβ PET imaging was carried out with a 20-minute acquisition, 50 minutes after intravenous injection of 200 MBq of 18F-NAV4694. Tau PET imaging was conducted with a 20-minute acquisition, 90 minutes after intravenous injection of 185 MBq of 18F-MK6240, on a separate day.
The Aβ PET scans were spatially normalized using CapAIBL (19) and the Centiloid (CL) scale was used to standardise the results (20, 21). The tau PET scans were spatially normalized using the MR-less CapAIBL PCA-based approach (22) and scaled using the cerebellar cortex as the reference region. We estimated the 18F-MK6240 standardized uptake value ratio (SUVR) for two in-house composite regions of interest (ROI): 1) mesial temporal (Me) ROI, comprising entorhinal cortex, amygdala, hippocampus, and parahippocampus and 2) temporoparietal (Te) ROI, comprising inferior and middle temporal, fusiform, supramarginal and angular gyri, posterior cingulate/ precuneus, superior and inferior parietal, and lateral occipital cortex (23). Tau 18F-MK6240 SUVR was also estimated in a standard meta-temporal composite region (MetaT), comprising FreeSurfer derived entorhinal cortex, parahippocampus, amygdala, inferior temporal, fusiform, and middle temporal cortex ROI (24).
A CL value of 20 was selected as the threshold to discriminate Aβ positive (A+) vs Aβ negative (A-) PET scans (25). Previously reported thresholds based on the 95th percentile of A- CU were used to discriminate tau positive (T+) from tau negative (T-) PET scans — 1.18 SUVR for Me (T+Me), 1.24 SUVR for Te (T+Te) (26) and 1.19 SUVR for MetaT (T+MetaT) (27).
p217+tau Assay
Fasted blood sampling was undertaken 2.3 ± 7 months from the time of Aβ PET scan and 1.9±2 months from the tau PET scan and on the same day as the baseline neuropsychological assessment. Plasma from K2-EDTA tubes (7.5 mL S-monovette 01.1605.008, Sarstedt), containing prostaglandin E1 (33 ng/mL of whole blood, Sapphire Biosciences) to prevent platelet activation, was centrifuged at room temperature at 200g for 10 mins to collect platelet-rich plasma. Then, it was centrifuged at 800g for 10 mins to provide plasma that was snap frozen within 2 hr of collection, following which it was stored in vapour phase liquid nitrogen prior to shipping on dry ice from Australia to Janssen R&D, La Jolla, CA, USA. The plasma p217+tau assay was performed on a Single Molecule Array (Simoa®) HD-X platform, blinded to all subject data using the technique previously described (11). Data analysis was performed by AIBL investigators. Optimal p217+tau thresholds to detect participants who showed any degree of cognitive decline on each of the cognitive measures between baseline and follow-up were derived using Youden’s Index. Binary p217+tau (pT-/pT+) groups were also created using previously reported thresholds for detection of A+ PET (12). Values for prevalence and predictive value of p217+tau positivity were also obtained by setting a sensitivity of 80% to detect PET A+ or T+.
Statistical Analysis
Statistical analyses were completed using python version 3.9.12. In the CI group, one participant had a plasma p217+tau concentration of 3.6x the mean (745.41 fg/ml) and therefore was excluded from further analysis as an outlier. Demographic difference between groups were assessed using independent t test for continuous data and Pearson’s χ2 test of independence for categorical variables (gender and APOE ε4 status). To calculate annual rate of cognitive change for each individual, a linear model was fit to each participant’s MMSE, CDR-SB, or AIBL-PACC over two to four timepoints independently and the regression slopes were derived for each cognitive measure separately. A regression slope threshold of zero was used to classify participants as cognitive decliners vs non-decliners: participants with negative regression slope for MMSE or AIBL-PACC, or positive slope for CDR-SB were considered as cognitive decliners. The correlation between baseline biomarkers — plasma p217+tau, tau PET, and Aβ PET— and rate of change in MMSE, CDR-SB, or AIBL-PACC was evaluated using Spearman’s rank-based correlation (Spearman’s Rho [ρ]). To evaluate the association of baseline biomarkers with change in cognition, after adjusting for baseline age, gender, APOE ε4 status, and years of education as covariates, multivariable linear regression models were used. Standardised beta coefficients, β, and their associated p values are reported. The areas under the receiver operating characteristic curves (AUC) were used to assess the performance of baseline p217+tau in discriminating cognitive decliners from those who remained cognitively stable. AUC, specificity, sensitivity, and positive predictive and negative predictive values (PPV and NPV, respectively) are reported with bootstrapped 95% confidence intervals (shown in square brackets). PPV and NPV were not disease prevalence adjusted. The AUCs for plasma vs imaging biomarkers were compared using DeLong test.
Power analysis was performed using the following formula:
n refers to estimated sample size, σ2 to an estimate of the population variance, and μ1-μ2 to the difference in mean values of both samples. P represents the power of the test, and α the significance level. Sample sizes were estimated — with 90% power to detect 30% reduction of decline on CDR-SB, two-sided 0.05 test— for either no screening, screening with blood test for participants who are pT+, or screening with PET for T+Me, T+Te, T+MetaT, or A+.
Results
Participant demographics
The 203 participants included 153 CU and 50 CI individuals (29 MCI and 21 mild dementia). Demographic characteristics of the participants are presented in Table 1.
Differences between cognitively unimpaired and cognitively impaired groups. Values presented as mean ± SD for all variables except Sample size, Gender and APOE ε4. CI cognitively impaired at baseline; CU cognitively unimpaired at baseline; unc. uncorrected; MMSE mini-mental state examination; CDR-SB clinical dementia rating sum of boxes; AIBL-PACC AIBL Preclinical Alzheimer’s disease Cognitive Composite; SD Standard deviation
Association of p217+tau versus PET biomarkers with prospective cognitive decline
Spearman’s ρ between baseline biomarkers and change in MMSE, CDR-SB or AIBL-PACC are shown in Figure 1. After controlling for baseline age, gender, APOE ε4, and years of education as covariates, results of separate multivariable linear regressions applied to the CU and CI groups are reported below.
Association of baseline plasma p217+tau concentration (fg/ml), tau MetaT SUVR and Aβ CL with annual rate of cognitive decline in the CI and CU groups. MMSE and CDR-SB were used as measures of cognition in the CI group and AIBL-PACC was used as a measure of cognition in the CU group. ATN profiles are based on neuroimaging biomarkers of Aβ and tau, with 20 CL and 1.19 SUVRMetaT as thresholds of A and T positivity, respectively. CI cognitively impaired at baseline; CU cognitively unimpaired at baseline; MMSE mini-mental state examination; CDR-SB clinical dementia rating sum of boxes; AIBL-PACC AIBL Preclinical Alzheimer’s disease Cognitive Composite; ρ Spearman’s rank correlation
In the CI group, plasma p217+tau concentration was a significant predictor of rate of change in MMSE (β = -0.55, p < 0.001) and CDR-SB (β = 0.61, p < 0.001). Association of p217+tau with cognitive decline had a comparable effect size to that for Aβ CL (β = -0.48, p = 0.002 for MMSE as the dependent variable; β = 0.43, p = 0.004 for CDR-SB as the dependent variable), and tau MetaT SUVR (β = -0.62, p < 0.001 for MMSE; β = 0.65, p < 0.001 for CDR-SB). Association of the covariates with the rate of change in cognition did not reach statistical significance in any of the models.
In the CU group, the regression coefficients were much smaller and not significant for plasma p217+tau (β = -0.1, p = 0.25) or Aβ CL (β = -0.11, p = 0.21) for annual rate of change in AIBL-PACC. The association of tau MetaT SUVR was small but statistically significant (β = -0.22, p = 0.008). Among the covariates, age had a significant association with change in AIBL-PACC (β = -0.18, p = 0.034) only in the model including p217+tau.
Figure 2 displays the cognitive trajectories for both the CI and CU groups, stratified by biomarker status (based on previously reported thresholds (See Methods section)), further illustrating a better performance in CI of all biomarkers in separating declining vs stable cognitive trajectories, than the CU group. It also illustrates a better performance of tau PET (specifically Me and MetaT ROIs) in separating declining vs stable cognitive trajectories in the CU group, than Aβ PET and p217+tau.
Cognitive trajectories in the CI (upper panel) and CU (lower panel) groups. Thresholds for biomarker status based on previously reported cut-off points (See Methods). The black dotted line indicates the regression line fitted to the whole cohort. Red line fitted to biomarker positive group. Blue line fitted to biomarker negative group. Time 0 represents baseline. Shaded areas represent bootstrapped 90% confidence Interval. First column is using p217+tau, next column is mesial temporal tau PET, then temporoparietal tau PET, then meta-temporal tau PET, and final column is amyloid PET. CI cognitively impaired at baseline; CU cognitively unimpaired at baseline; CDR-SB clinical dementia rating sum of boxes; PACC AIBL Preclinical Alzheimer’s disease Cognitive Composite; Me mesial temporal ROI; Te temporoparietal ROI; MetaT meta-temporal composite region.
Performance of p217+tau in detecting cognitive decliners
In the CI group, using the status of MMSE slope as the outcome measure, plasma p217+tau discriminated cognitive decliners from cognitively stable participants with an AUC of 0.74 [0.62 – 0.85]. Youden’s Index provided a cut-off point of 124.6 fg/ml (Figure 3, left panel) which yielded a sensitivity of 0.78 [0.50 – 0.93], specificity of 0.65 [0.53 – 0.96], PPV of 0.71 [0.62 – 0.94], and NPV of 0.71 [0.56 – 0.89]. Using the status of CDR-SB slope as the outcome measure, p217+tau discriminated cognitive decliners from stable participants with an AUC of 0.83 [0.73 – 0.92]. The Youden Index threshold was 131.1 fg/ml (Figure 3, right panel) giving sensitivity of 0.75 [0.57 – 1.0], specificity of 0.77 [0.48 – 0.96], PPV of 0.80 [0.66 – 0.96], and NPV of 0.71 [0.60 – 1.0]. Supplementary Table 1 presents a comparison of AUCs, for discriminating cognitive decliners from cognitively stable participants in the CI group, between p217+tau, tau PET (Me, Te and MetaT ROIs) and Aβ PET using DeLong test. Though the AUC was higher for plasma p217+tau than the PET measures, this was not significant.
Among CU only, plasma p217+tau was not able to discriminate cognitive decliners assessed by AIBL-PACC from cognitively stable individuals. The AUC was 0.53 [0.45 – 0.61], with a Youden threshold of 104.5 fg/ml yielding a sensitivity of 0.38 [0.12 – 0.78], specificity of 0.75 [0.38 – 0.98], PPV of 0.62 [0.53 – 0.85] and NPV of 0.52 [0.46 – 0.64].
Plasma p217+tau vs MMSE and CDR-SB slope. The vertical dashed lines display the plasma p217+tau Youden threshold (124.6 fg/ml for the left panel and 131.1 fg/ml for the right panel). The horizontal dashed lines represent the threshold of zero for dichotomizing cognitive slopes to positive and negative status. ATN profiles are based on neuroimaging biomarkers of Aβ and tau, with 20 CL and 1.19 SUVRMetaT as thresholds of A and T positivity, respectively. MMSE mini-mental state examination; CDR-SB clinical dementia rating sum of boxes.
Enrichment of trial populations using p217+tau versus PET biomarkers
The average rate of cognitive decline measured by AIBL-PACC in the CU group (dotted line in Figure 2, CU) was -0.01 ± 0.33 and was not significantly different to those who were above or below the p217+tau threshold. Therefore, power analysis was only performed in the CI group. Table 2a presents the Mean ± SD of CDR-SB slope and estimated sample size for a trial to detect 30% slowing of decline in CDR-SB for no biomarker screening and screening for plasma pT+, and PET T+Me, T+Te, T+MetaT and A+ participants. Table 2b presents the estimated cost of screening using each biomarker. Table 2c shows the estimated cost when pre-screening with plasma p217+tau followed by PET scan of the pT+ individuals to find T+ or A+ participants.
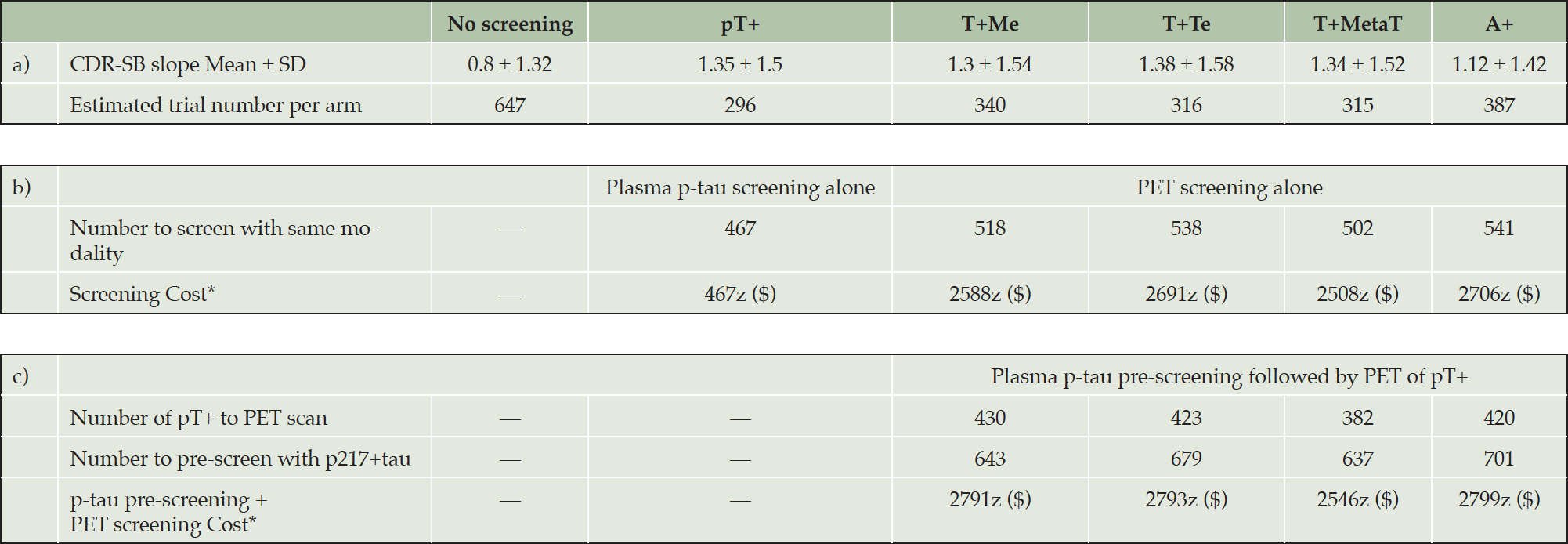
Table 2. Trial size & cost estimation for participants with cognitive impairment (MCI or mild dementia)
Binary p217+tau (pT-/pT+), tau (T-/T+) and Aβ (A-/A+) groups based on previously reported established cut-off points: 126.7 fg/ml for pT+, 1.18 SUVR for T+Me, 1.24 SUVR for T+Te, 1.19 SUVR for T+MetaT and 20 CL for A+ a) Estimated trial number per arm power analysis with primary end point of 30% slowing decline on CDR-SB in CI over 2 years with 90% power, 0.05 alpha. b) Number to screen with same modality estimated based on observed prevalence: 0.63 for pT+, 0.66 for T+Me, 0.59 for T+Te, 0.63 for T+MetaT, and 0.72 for A+. c) p217+tau threshold set to 80% sensitivity to detect A+ or T+. Number to pre-screen with p217+tau and Number of pT+ to PET scan estimated based on observed prevalence and predictive value of p217+tau positivity. Observed prevalence of pT+ for T+Me 0.67, for T+Te 0.62, for T+MetaT 0.60 and for A+ 0.60. PPV of p217+tau for T+Me 0.79, for T+Te 0.75, for T+MetaT 0.83 and for A+ 0.92. CDR-SB Clinical Dementia Rating Sum of Boxes. *Cost per trial arm, assuming a PET scan costs 5 times a blood test (cost of blood test: z, cost of PET scan: 5z)
Plasma p217+tau as a screening tool
Based on the thresholds used in this study, plasma pT+ individuals had a rate of decline on CDR-SB (Mean ± SD: 1.35 ± 1.5) within a similar range to the PET T+ groups (Mean ± SD: 1.3 ± 1.54 for T+Me; 1.38 ± 1.58 for T+Te; 1.34 ± 1.52 for T+MetaT). The power analysis showed that, using previously published thresholds, screening pT+ CI participants into a two-year therapeutic trial, where the aim was to slow cognitive decline by 30%, led to similar sample size requirements compared to screening using PET (Table 2a).
The observed prevalence in the CI cohort was 63% for pT+, 66% for T+Me, 59% for T+Te, 63% for T+MetaT, and 72% for A+. The Number to screen with same modality was estimated based on observed prevalence of biomarker positivity in the 172 CI with cross sectional data, that we have previously reported (12), that included the 50 CI with subsequent follow-up reported in this manuscript. In the following sections, we have assumed that a blood test (z) costs one fifth of a PET scan. The results show that using p217+tau alone to select CI participants for a clinical trial would translate to 81 to 83% lower biomarker test cost than participant selection by PET (Table 2b). At a PET to blood test cost ratio (a) of 5, 80% ((a-1)/a) of the reported cost-saving was a result of the lower cost of a blood test while the remaining 1-3% cost saving resulted from the slightly smaller cohort size required for a pT+ group.
Plasma p217+tau as a pre-screening tool prior to PET
The plasma p217+tau threshold was set at 80% sensitivity to detect PET positive cases for each PET measure and was 122.3 fg/ml for detecting T+Me, 128.9 fg/ml for T+Te, and 131.1 fg/ml for T+MetaT and A+. Number to pre-screen with p217+tau and Number of pT+ to PET scan were calculated using prevalence and predictive value of p217+tau positivity for T+Me (0.67, 0.79), T+Te (0.62, 0.75), T+MetaT (0.60, 0.83), and A+ (0.60, 0.92). The results showed that in a therapeutic trial recruiting PET A+ or T+ CI participants, p217+tau pre-screening would reduce the number of required PET scans and therefore cost by 17-24% but incur an additional cost of 25-26% for blood testing. Thus, p217+tau pre-screening followed by PET of pT+ individuals does not result in any cost-saving relative to direct screening using PET alone (Table 2c). This contrasts to a 26-38% biomarker test cost-saving in the CU group (Supplementary Table 2). Some level of biomarker test cost-saving could be achieved in CI participants if a plasma p217+tau test costs less than one fifth of a PET scan (Supplementary Table 3). However, this would likely be negated by the increase of approximately 25-30% in the total number of CI needed to screen for the study.
The aforementioned results are also reported for binary pT-/pT+, T-/T+, and A-/A+ groups created using a threshold from AUC analyses (threshold set using 80% sensitivity to detect a positive CDR-SB slope) in Supplementary Table 4 but again showed that pre-screening with p217+tau prior to PET was not cost saving.
Discussion
In a group of cognitively unimpaired individuals and MCI and mild dementia patients, we assessed the prognostic performance of a novel plasma-based biomarker, p217+tau, measured using Simoa. We investigated the role of p217+tau in predicting cognitive decline, as compared to neuroimaging biomarkers of Aβ and tau pathology. We also investigated the screening and pre-screening capability of plasma p217+tau in a therapeutic trial.
Both plasma p217+tau and neuroimaging biomarkers better predicted those who would cognitively decline in the cognitively impaired (CI) cohort compared to the cognitively unimpaired cohort. After adjusting for the effect in CI of age, sex, APOE ε4 status and years of education, higher levels of p217+tau were significantly associated with higher annual rate of change in cognition. However, in the CU group, increasing levels of tau PET MetaT SUVR, but not plasma p217+tau nor Aβ PET CL, were significantly associated with faster rate of cognitive decline over a two-year period.
Groot et al. (28) showed that baseline plasma p217+tau had a moderate association (r = −0.45) with annual change in MMSE although the reported association was across a mix of CU and MCI participants and did not include dementia stage AD. Our study found a Spearman correlation of -0.55 in CI between p217+tau and annual change in MMSE. The weaker associations found between p217+tau and cognition in our CU vs CI group accords with previous reports of other plasma p-tau isoforms and high sensitivity immunoassays. The performance of longitudinal change in p-tau217 (Lilly MSD assay) in predicting MMSE slope in MCI (β =-0.35) was better than that in CU (β = -0.15) (29). Therriault et al. (30) also reported a much lower β estimate of -0.01 for the association between baseline plasma p-tau181 status using Simoa® and longitudinal decline in memory in CU participants while the association between the same variables in the MCI group gave a β estimate of -0.12.
Our results also showed in CI that in 71% of those with a plasma p217+tau concentration > 124.6 fg/ml there was decline on MMSE within 2.4 years. A concentration > 131.1 fg/ml was associated with decline on CDR within 2.4 years in 80% of pT+ individuals. These thresholds approximated the previously reported Youden’s threshold of p217+tau (126.7 fg/ml) for discriminating both Aβ+ from Aβ- PET and tau+ from tau- PET in CI participants (12).
We also investigated the suitability of plasma p217+tau as a stand-alone screening measure for a therapeutic trial designed to slow cognitive decline by 30%. Based on the Alzheimer’s Association appropriate use recommendations for blood biomarkers (31), the required PPV for valid prediction of presence of AD pathological changes is considered to be > 90%. Doré et al. (12) previously reported that in CI individuals, plasma p217+tau has a PPV of 92% for positive Aβ PET scan at a cut-off point of 126.7 fg/ml (pT+). Using this threshold to define the pT+ group in our study, we assumed pT+ participants do not need to undergo a PET scan to confirm a positive AD status. Hence, screening the required number of CI individuals with p217+tau rather than PET would be cost-effective with an 81-83% reduction in biomarker test costs. In contrast, in CU individuals, given that plasma p217+tau (at Youden’s Index threshold of 100.3 fg/ml) has a high NPV (90%) but low PPV (57%) for positive Aβ PET scan (12), p217+tau screening alone is not appropriate as a stand-alone screen for preclinical AD, unless the p217+tau threshold is set to 90% PPV for A+ but this would increase the number needed to screen by >400%.
Next, the utility of plasma p217+tau as a pre-screening tool was investigated. In our study, we assumed a commercial PET scan would cost 5 times a commercial p-tau blood test and conducted this analysis in both the CU and CI cohorts, for both Aβ and tau PET at different ROIs. With this assumption and prevalence and PPV values derived from our sample of 397 participants, p217+tau pre-screening returned substantial cost-saving in the CU (26-38% test cost reduction), but not in the CI group. Our findings in regard to CU are in agreement with a previous study by Karikari, et al. (3). They reported that using blood p-tau181 pre-screening of asymptomatic participants followed by Aβ PET in only those with a high p-tau181 level would provide a 58% cost-saving— assuming a PET scan cost sixty times a blood test — compared with the traditional approach of PET screening all potential participants.
A major strength of this study was the inclusion of participants with plasma p217+tau, tau PET, Aβ PET, and baseline and follow-up neuropsychological assessment data. This allowed comparison of three biomarkers with regard to their association with longitudinal cognitive trajectories in both early and later stages of the AD continuum. However, the study has several noteworthy limitations. Firstly, we followed the participants for an average of 2.4 years. Longer follow-up is required in CU to determine the predictive value of plasma p217+tau for cognitive decline. Secondly, given the relatively small sample size in the CI group comprising MCI and AD participants and lack of a validation cohort, the findings of this study need to be replicated in a larger independent cohort of individuals; particularly more data is needed to replicate > 90% PPV of p217+tau for AD pathological change, to provide further support for its use as a stand-alone biomarker for trial inclusion. Lastly, the participants in this study were typical of those recruited into previous AD therapeutic trials. Therefore, the findings may not be generalizable to trials recruiting a population with greater ethnic and health diversity or who have had less rigorous clinical and cognitive assessment compared to that undertaken prior to inclusion in this study. The cost calculations are based on an amyloid PET positive prevalence of 72% in the CI cohort. As expected, the prevalence was lower in MCI (55%) than AD (80%) so the finding of little or no test cost saving in CI may not apply to a pure MCI population. We also set the plasma p217+tau sensitivity to 80% detection of positive PET. A more sensitive assay may alter the cost calculations in favour of blood pre-screening.
Conclusion
These data suggests that substantial cost reduction can be achieved using plasma p217+tau alone to select participants with cognitive impairment in the MCI/mild AD range for a clinical trial designed to slow cognitive decline by 30% over two years, compared to participant selection using PET. Using p217+tau for pre-screening in MCI and mild dementia prior to PET is unlikely to be cost-effective. Any test cost saving may be offset by the increase in the number of participants needed to screen necessitating a significant increase in the duration of the recruitment phase or use of more trial sites. In contrast, in the cognitively unimpaired population, unlike tau PET, p217+tau was not able to predict cognitive decline over two years but provided significant cost-saving if used as a pre-screening measure for A+ or T+ PET. These findings require replication in larger cohorts.
Acknowledgments: Some data used in this research article were obtained from the Australian Imaging Biomarkers and Lifestyle flagship study of aging (AIBL), funded by the Commonwealth Scientific and Industrial Research Organization (CSIRO), National Health and Medical Research Council (NHMRC), and other participating institutions. Some data were obtained from the Australian Dementia Network (ADNeT) that receives funding support from the NHMRC. AIBL researchers are listed on the website www.aibl.csiro.au. The authors thank those who participated in the study, as well as their families.
Funding: The research was supported by the Australian Federal Government through NHMRC grants APP1132604, APP1140853 and APP1152623 and by a grant from Enigma Australia. Janssen Pharmaceuticals paid a commercial data access fee to the AIBL study of ageing. Janssen Pharmaceuticals performed the p217+tau measurements but did not perform the data analysis. The co-authors employed by Janssen did provide comments on the manuscript and Janssen provided approval for submission of the manuscript. The other sponsors had no role in the design and conduct of the study, in the collection, analysis, and interpretation of data, or in the preparation of the manuscript. Open Access funding enabled and organized by CAUL and its Member Institutions.
Conflict of interest: Christopher C. Rowe has received research grants from NHMRC, Enigma Australia, Biogen, Eisai and Abbvie. He is on the scientific advisory board for Cerveau Technologies and has consulted for Prothena, Eisai, Roche, and Biogen Australia. Victor L. Villemagne is and has been a consultant or paid speaker at sponsored conference sessions for Eli Lilly, Life Molecular Imaging, GE Healthcare, IXICO, Abbvie, Lundbeck, Shanghai Green Valley Pharmaceutical Co Ltd, and Hoffmann La Roche. Ashley I. Bush is a shareholder in Alterity Ltd, Cogstate Ltd and Mesoblast Ltd. He is a paid consultant for, and has a profit share interest in Collaborative Medicinal Development Pty Ltd. He has received lecture fees from Biogen P/L. Paul Maruff was a full-time employee of Cogstate. Ziad S. Saad, Gallen Triana-Baltzer, Randy Slemmon and Hartmuth C. Kolb are employees of Janssen Pharmaceuticals. The other authors did not report any conflict of interest.
Ethical standards: The study was approved by the Austin Health Human Research Ethics Committee and all participants gave written consent.
Open Access: This article is distributed under the terms of the Creative Commons Attribution 4.0 International License (http://creativecommons.org/licenses/by/4.0/), which permits use, duplication, adaptation, distribution and reproduction in any medium or format, as long as you give appropriate credit to the original author(s) and the source, provide a link to the Creative Commons license and indicate if changes were made.
References
1. Jack Jr CR, Bennett DA, Blennow K, et al. NIA-AA research framework: toward a biological definition of Alzheimer’s disease. Alzheimers Dement 2018;14(4):535-562, doi: https://doi.org/10.1016/j.jalz.2018.02.018
2. Karikari TK, Ashton NJ, Brinkmalm G, et al. Blood phospho-tau in Alzheimer disease: analysis, interpretation, and clinical utility. Nat Rev Neurol 2022;18:400–418, doi:https://doi.org/10.1038/s41582-022-00665-2
3. Karikari TK, Benedet AL, Ashton NJ, et al. Diagnostic performance and prediction of clinical progression of plasma phospho-tau181 in the Alzheimer’s Disease Neuroimaging Initiative. Mol Psychiatry 2021;26(2):429-442, doi:https://doi.org/10.1038/s41380-020-00923-z
4. Mielke MM, Hagen CE, Xu J, et al. Plasma phospho-tau181 increases with Alzheimer’s disease clinical severity and is associated with tau-and amyloid-positron emission tomography. Alzheimers Dement 2018;14(8):989-997, doi: https://doi.org/10.1016/j.jalz.2018.02.013
5. Palmqvist S, Janelidze S, Quiroz YT, et al. Discriminative accuracy of plasma phospho-tau217 for Alzheimer disease vs other neurodegenerative disorders. Jama 2020;324(8):772-781, doi: https://doi.org/10.1001/jama.2020.12134
6. Bayoumy S, Verberk IM, den Dulk B, et al. Clinical and analytical comparison of six Simoa assays for plasma P-tau isoforms P-tau181, P-tau217, and P-tau231. Alzheimers Res Ther 2021;13(1):1-15, doi:https://doi.org/10.1186/s13195-021-00939-9
7. Ashton NJ, Pascoal TA, Karikari TK, et al. Plasma p-tau231: a new biomarker for incipient Alzheimer’s disease pathology. Acta Neuropathol 2021;141(5):709-724, doi: https://doi.org/10.1007/s00401-021-02275-6
8. Janelidze S, Mattsson N, Palmqvist S, et al. Plasma P-tau181 in Alzheimer’s disease: relationship to other biomarkers, differential diagnosis, neuropathology and longitudinal progression to Alzheimer’s dementia. Nat Med 2020;26(3):379-386, doi: https://doi.org/10.1038/s41591-020-0755-1
9. Thijssen EH, La Joie R, Wolf A, et al. Diagnostic value of plasma phosphorylated tau181 in Alzheimer’s disease and frontotemporal lobar degeneration. Nat Med 2020;26(3):387-397, doi:https://doi.org/10.1038/s41591-020-0762-2
10. Xia Y, Prokop S , Giasson BI. “Don’t Phos Over Tau”: recent developments in clinical biomarkers and therapies targeting tau phosphorylation in Alzheimer’s disease and other tauopathies. Mol Neurodegener 2021;16(1):1-19, doi: https://doi.org/10.1186/s13024-021-00460-5
11. Triana-Baltzer G, Moughadam S, Slemmon R, et al. Development and validation of a high-sensitivity assay for measuring p217+ tau in plasma. Alzheimers Dement: Diagn Assess Dis Monit 2021;13(1):e12204, doi: https://doi.org/10.1002/dad2.12204
12. Doré V, Doecke JD, Saad ZS, et al. Plasma p217+ tau versus NAV4694 amyloid and MK6240 tau PET across the Alzheimer’s continuum. Alzheimers Dement: Diagn Assess Dis Monit 2022;14(1):e12307, doi: https://doi.org/10.1002/dad2.12307
13. Fowler C, Rainey-Smith SR, Bird S, et al. Fifteen years of the australian imaging, biomarkers and lifestyle (AIBL) study: progress and observations from 2,359 older adults spanning the spectrum from cognitive normality to Alzheimer’s disease. J Alzheimers Dis Rep 2021;5(1):443-468, doi: https://doi.org/10.3233/ADR-210005
14. Petersen RC, Caracciolo B, Brayne C, Gauthier S, Jelic V , Fratiglioni L. Mild cognitive impairment: a concept in evolution. J Intern Med 2014;275(3):214-28, doi: https://doi.org/10.1111/joim.12190
15. McKhann GM, Knopman DS, Chertkow H, et al. The diagnosis of dementia due to Alzheimer’s disease: recommendations from the National Institute on Aging-Alzheimer’s Association workgroups on diagnostic guidelines for Alzheimer’s disease. Alzheimers Dement 2011;7(3):263-9, doi: https://doi.org/10.1016/j.jalz.2011.03.005
16. Ellis KA, Bush AI, Darby D, et al. The Australian Imaging, Biomarkers and Lifestyle (AIBL) study of aging: methodology and baseline characteristics of 1112 individuals recruited for a longitudinal study of Alzheimer’s disease. Int Psychogeriatr 2009;21(4):672-687, doi: https://doi.org/10.1017/S1041610209009405
17. Morris JC. Clinical dementia rating: a reliable and valid diagnostic and staging measure for dementia of the Alzheimer type. Int Psychogeriatr 1997;9(S1):173-176, doi: https://doi.org/10.1017/s1041610297004870
18. Donohue MC, Sperling RA, Salmon DP, et al. The preclinical Alzheimer cognitive composite: measuring amyloid-related decline. JAMA Neurol 2014;71(8):961-970, doi: https://doi.org/10.1001/jamaneurol.2014.803
19. Bourgeat P, Doré V, Fripp J, et al. Implementing the centiloid transformation for 11C-PiB and β-amyloid 18F-PET tracers using CapAIBL. Neuroimage 2018;183(387-393, doi: https://doi.org/10.1016/j.neuroimage.2018.08.044
20. Klunk WE, Koeppe RA, Price JC, et al. The Centiloid Project: standardizing quantitative amyloid plaque estimation by PET. Alzheimers Dement 2015;11(1):1-15. e4, doi: https://doi.org/10.1016/j.jalz.2014.07.003
21. Rowe CC, Jones G, Doré V, et al. Standardized expression of 18F-NAV4694 and 11C-PiB β-amyloid PET results with the Centiloid scale. J Nucl Med 2016;57(8):1233-1237, doi: https://doi.org/10.2967/jnumed.115.171595
22. Dore V, Bourgeat P, Burnham SC, et al. Ic-P-167: automated reporting of tau PET quantification on brain surface. Alzheimers Dement 2019;15(P131-P132, doi: https://doi.org/10.1016/j.jalz.2019.06.4282
23. Villemagne V, Dore V , Bourgeat P. The Tau MeTeR composites for the generation of continuous and categorical measures of tau deposits in the brain. J Mol Med Ther 2017;1(1):25-32
24. Jack Jr CR, Wiste HJ, Schwarz CG, et al. Longitudinal tau PET in ageing and Alzheimer’s disease. Brain 2018;141(5):1517-1528, doi: https://doi.org/10.1093/brain/awy059
25. Rowe C, Amadoru S, Dore V, et al. Correlation of amyloid PET in Centiloid units with neuropathological findings in Alzheimer’s disease. Society of Nuclear Medicine: 2018
26. Doré V, Krishnadas N, Bourgeat P, et al. Relationship between amyloid and tau levels and its impact on tau spreading. Eur J Nucl Med Mol Imaging 2021;48(7):2225-2232, doi: https://doi.org/10.1007/s00259-021-05191-9
27. Villemagne VL, Harada R, Dore V, et al. Assessing reactive astrogliosis with 18F-SMBT-1 across the Alzheimer’s disease spectrum. J Nucl Med 2022;63(10):1560-1569, doi:https://doi.org/10.2967/jnumed.121.263255
28. Groot C, Cicognola C, Bali D, et al. Diagnostic and prognostic performance to detect Alzheimer’s disease and clinical progression of a novel assay for plasma p-tau217. Alzheimers Res Ther 2022;14(1):1-12, doi: https://doi.org/10.1186/s13195-022-01005-8
29. Mattsson-Carlgren N, Janelidze S, Palmqvist S, et al. Longitudinal plasma p-tau217 is increased in early stages of Alzheimer’s disease. Brain 2020;143(11):3234-3241, doi: https://doi.org/10.1093/brain/awaa286
30. Therriault J, Benedet AL, Pascoal TA, et al. Association of plasma P-tau181 with memory decline in non-demented adults. Brain commun 2021;3(3):fcab136, doi:https://doi.org/10.1093/braincomms/fcab136
31. Hansson O, Edelmayer RM, Boxer AL, et al. The Alzheimer’s Association appropriate use recommendations for blood biomarkers in Alzheimer’s disease. Alzheimers Dement 2022, doi:https://doi.org/10.1002/alz.12756
© The Authors 2023